Originally I wanted to make an oversight of all ways the so called Dirac quantization condition is represented. That is why in the beginning of this post below you can find some stuff on the Dirac equation and the four solutions that come with that equation. Anyway, Paul Dirac once managed to factorize the Laplacian operator, that was needed because the Laplacian is part of the Schrödinger equation that gives the desired wave functions in quantum mechanics. Well I had done that too once upon a time in a long long past and I remembered that the outcome was highly surprising. As a matter of fact I consider this one of the deeper secrets of the higher dimensional complex numbers. Now I use a so called Wirtinger derivative; for example on the space of 3D complex numbers you take the partial derivatives into the x, y and z direction and from those three partial derivatives you make the derivative. And once you have that, if you feed it a function you simply get the derivative of such a function.
Now such a Wirtinger derivative also has a conjugate and the surprising result is that if you multiply such a Wirtinger derivative against it’s conjugate you always get either the Laplacian or in the case of the 3D complex numbers you get the Laplacian multiplied by the famous number alpha.
That is a surprising result because if you multiply an ordinary 3D number X against it’s conjugate you get the equation of a sphere and a cone like thing. But if you do it with parital differential operators you can always rewrite it into pure Laplacians so there the cones and spheres are the same things…
In the past I only had it done on the space of 3D numbers so I checked it for the 4D complex numbers and in about 10 minutes of time I found out it also works on the space of 4D complex numbers. So I started writing this post and since I wanted to build it slowly up from 2D to 4D complex numbers it grew longer than expected. All in all this post is 15 pictures long and given the fact that people at present day do not have those long timespan of attention anymore, may be it is too long. I too have this fault, if you hang out on the preprint archive there is just so much material that often after only five minutes of reading you already go to another article. If the article is lucky, at best it gets saved to my hard disk and if the article has more luck in some future date I will read it again. For example in the year 2015 I saved an article that gave an oversight about the Dirac quantization condition and only now in 2020 I looked at it again…
The structure of this post is utterly simple: On every complex space (2D, 3D and 4D) I just give three examples. The examples are named example 1, 2 and not surprising I hope, example 3. These example are the same, only the underlying space of complex numbers varies. In each example number 1 I define the Wirtinger derivative, in example 2 I take the conjugate while in the third example on each space I multiply these two operators and rewrite the stuff into Laplacians. The reason this post is 15 pictures long lies in the fact that the more dimensions you have in your complex numbers the longer the calculations get. So it goes from rather short in the complex plane (the 2D complex numbers) to rather lengthy in the space of 4D complex numbers.
At last I would like to remark that those four simultanious solutions to the Dirac equation it once more shouts at your face: electrons carry magnetic charge and they are ot magnetic dipoles! All that stuff like the Pauli matrices where Dirac did build his stuff upon is sheer difficult nonsense: the interaction of electron spin with a magnetic field does not go that way. The only reason people in the 21-th century think it has some merits is because it is so complicated and people just loose oversight and do not see that it is bogus shit from the beginning till the end. Just like the math professors that neatly keep themselves stupid by not willing to talk about 3D complex numbers. Well we live in a free world and there are no laws against being stupid I just guess.
Enough of the blah blah blah, below are the 15 pictures. And in case you have never ever heard about a thing known as the Wirtinger derivative, try to understand it and may be come back in five or ten years so you can learn a bit more…
As usual all pictures are 550×775 pixels in size.
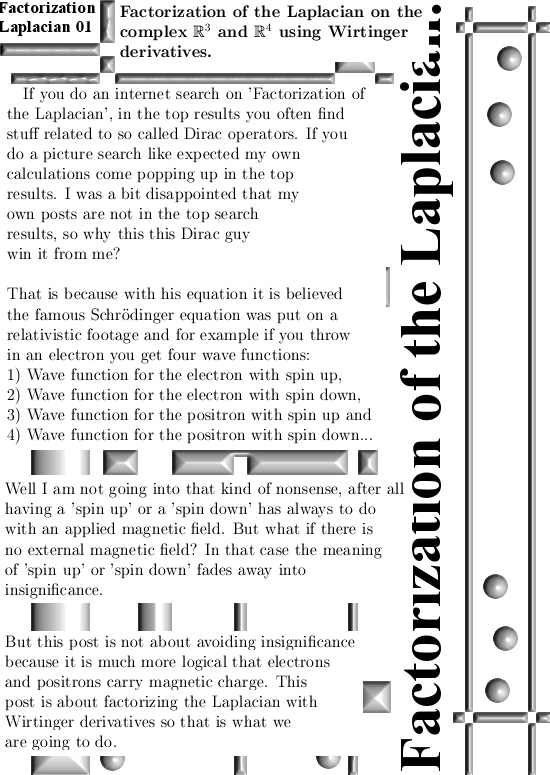
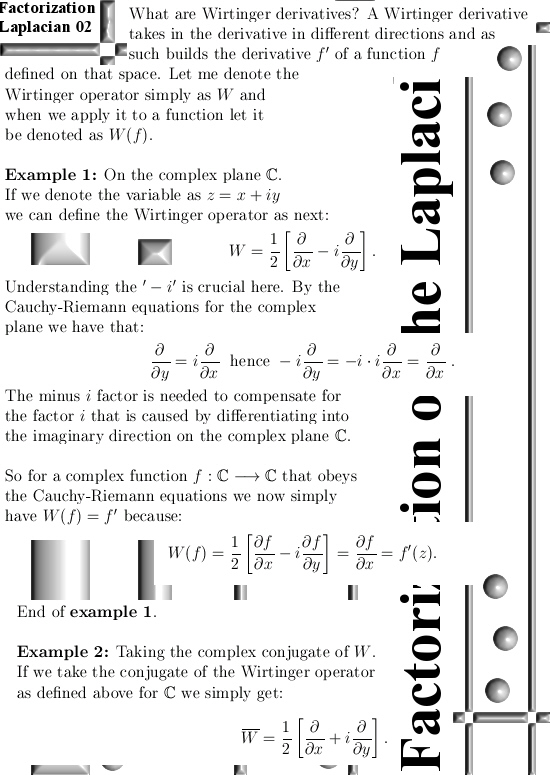
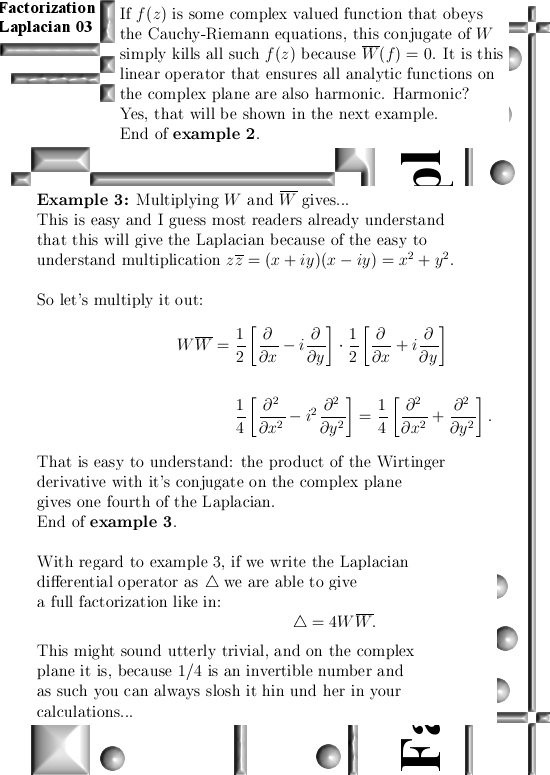
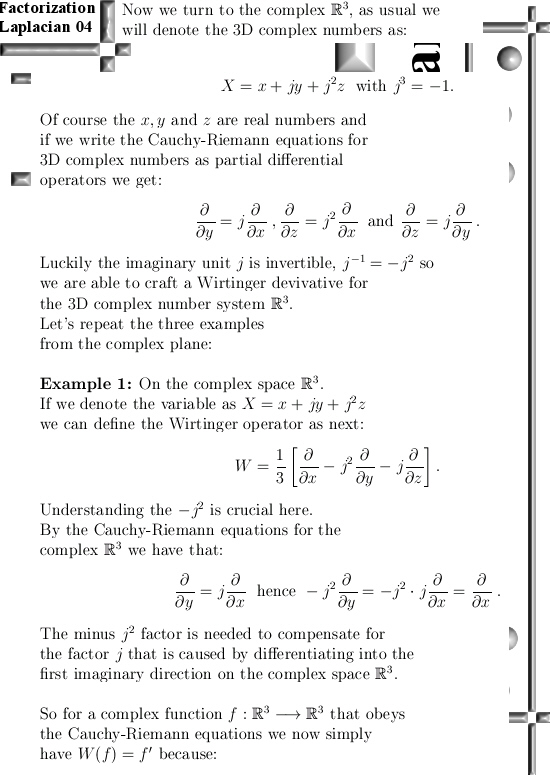
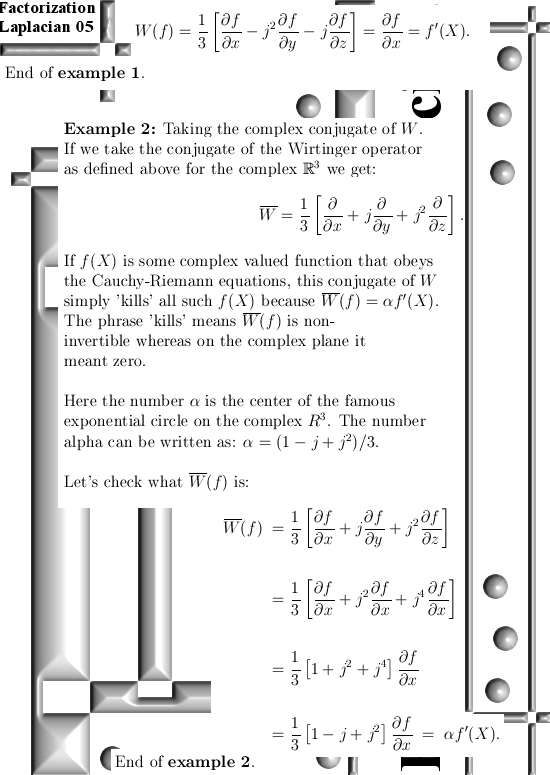
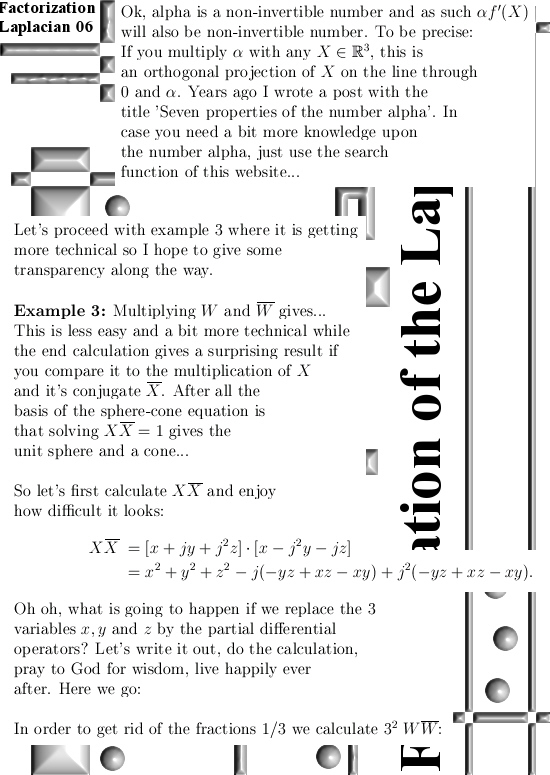
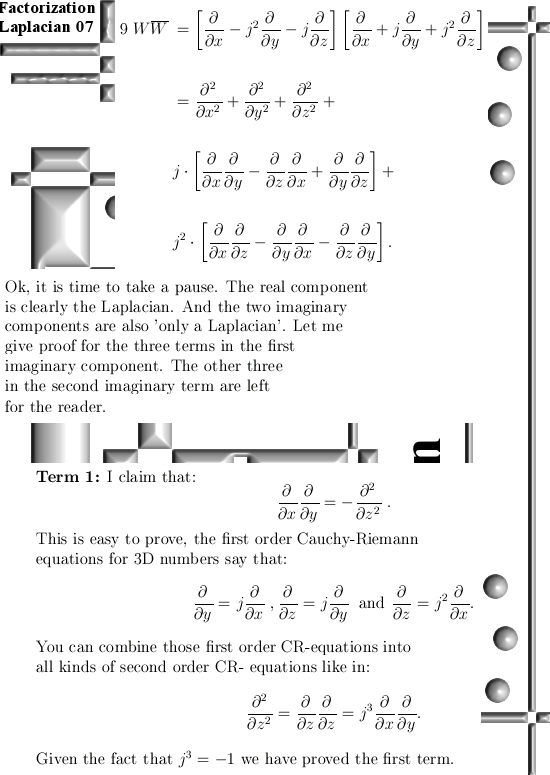
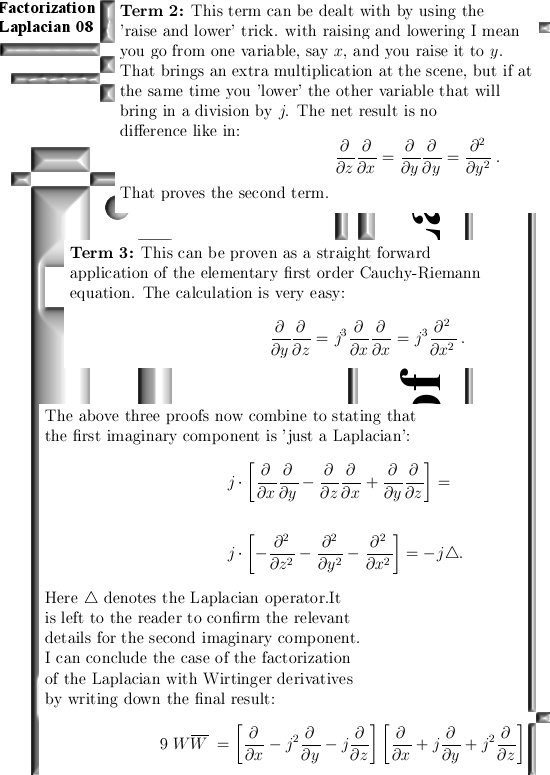
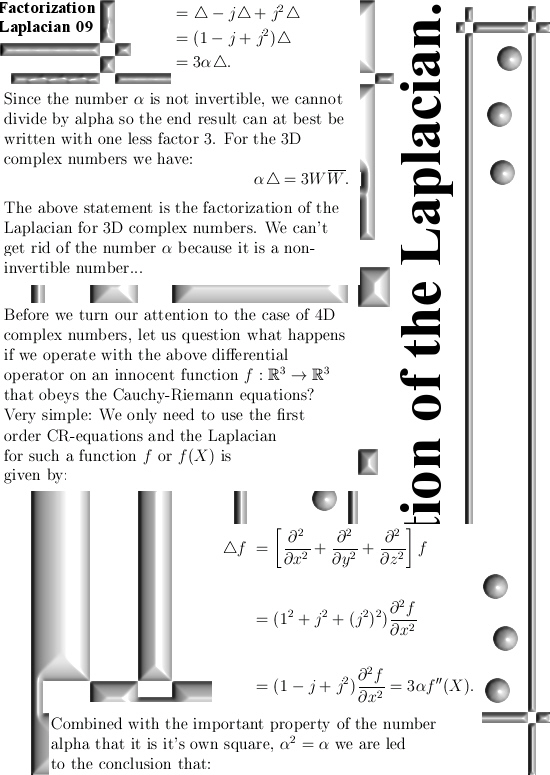
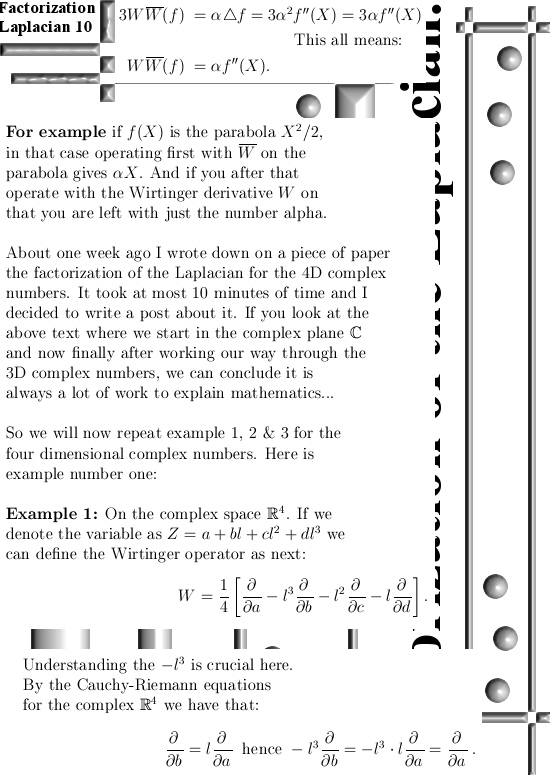
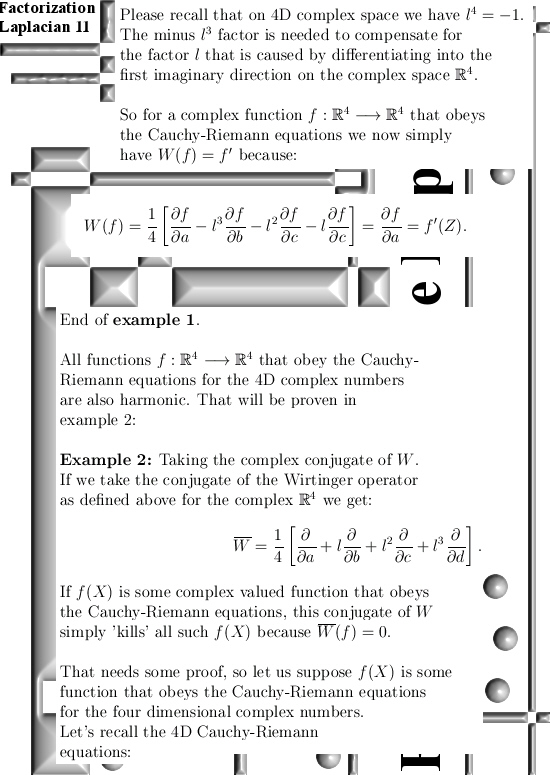
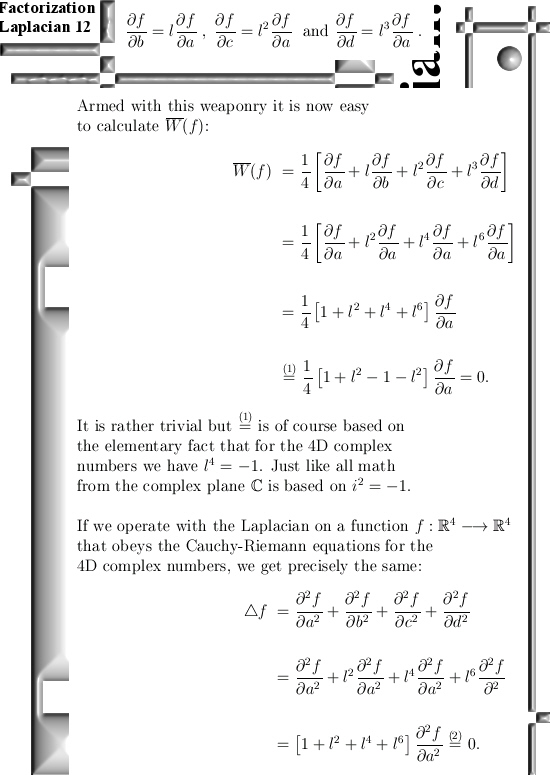
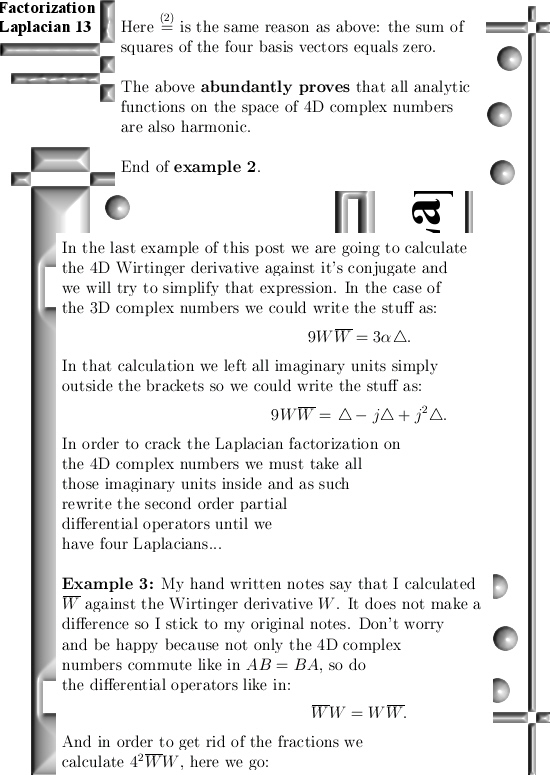
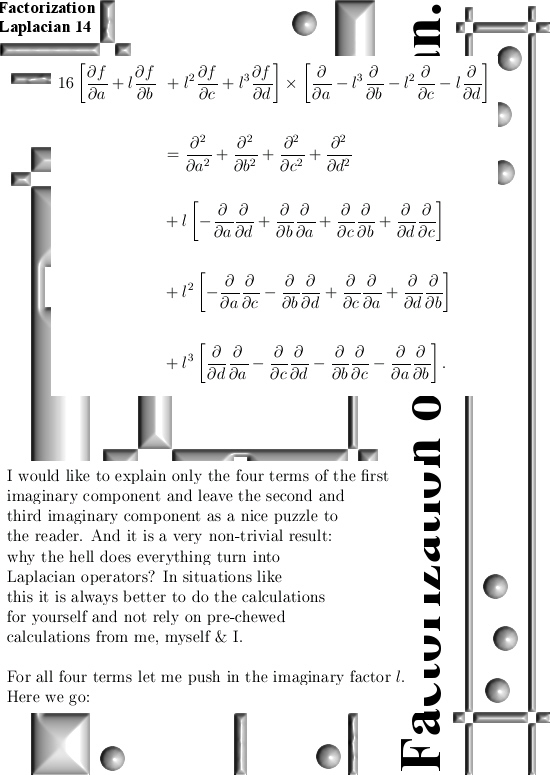
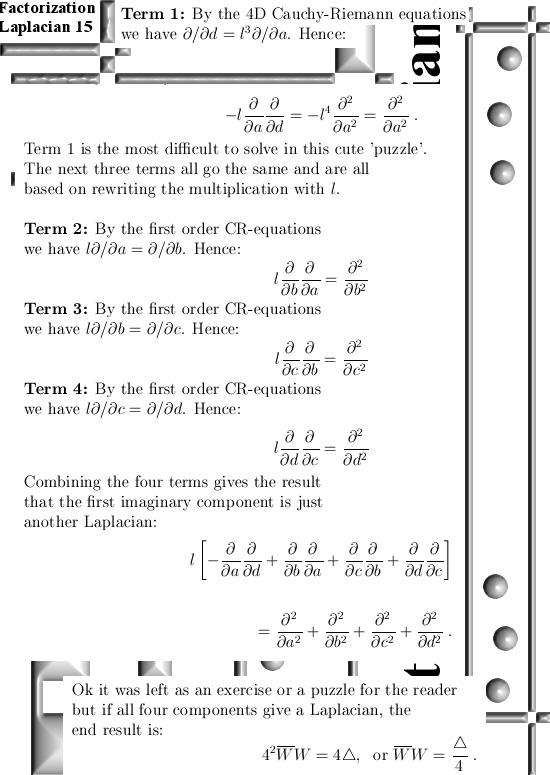
Oh oh the human mind and learning new things. If a human brain learns new things like Cauchy-Riemann equations or the above factoriztion of the Laplacian, a lot of chages happen in the brain tissue. And it makes you tired and you need to sleep…
And when you wake up, a lot of people look at their phone and may be it says: Wanna see those new pictures of Miley Cyrus showing her titties? And all your new learned things turn into insignificance because in the morning what is more important compared to Miley her titties?
Ok my dear reader, you are at the end of this post. See you in the next post.