This is a very short post, in it I even joke this is done in “Tik Tok style”. It is about finding the eigenvalues for an arbitray elliptic complex number. Such numbers have matrix representations and as such they have also eigenvalues that live in the ordinary complex plane.
Here the elliptic plane is the same as we always studied the last couple of months, it is ruled by the imaginary unit i via i^2 = -1 + i. If you need the eigenvalues of such a number, instead of going through the calculation for eigenvalues every time, with the eigenvalue functions you just substitute it in and it spits out the two eigenvalues.
To be honest I did not explain in detail why it works, I hope it is rather obvious. Take for example two commuting (square) matrices A and B. They have the same eigen vectors (because they commute) and as such it is very easy to find the eigenvalues of any linear combination of A and B.
The post itself is only two pictures long and I included a third picture that I used on the other website. Beside a female robot the third picture contains another factorization of the equation of the ellipse that is the determinant of the matrix representations.
So this equation for the ellipse can be factored on the standard complex plane and also on the elliptic complex plane. The interesting detail is of course that on the elliptic complex plane you have integer coefficients while on the standard complex plane this is impossible.
Basically the eigenvalue funtions are both a map from the elliptic complex plane to the ordinary complex plane. If it was made by a professional math professor he or she would likely call it an isomorphism but I name them eigenvalue functions. In the past I also made them for the 3D complex and circular numbers and of course for the 4D complex numbers that were under study years ago.
Enough of the introdutionary talk, lets go:
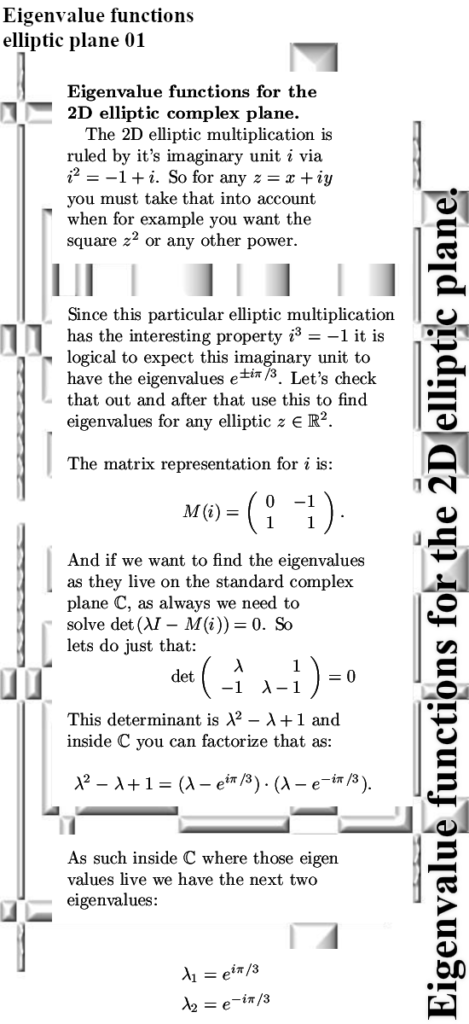
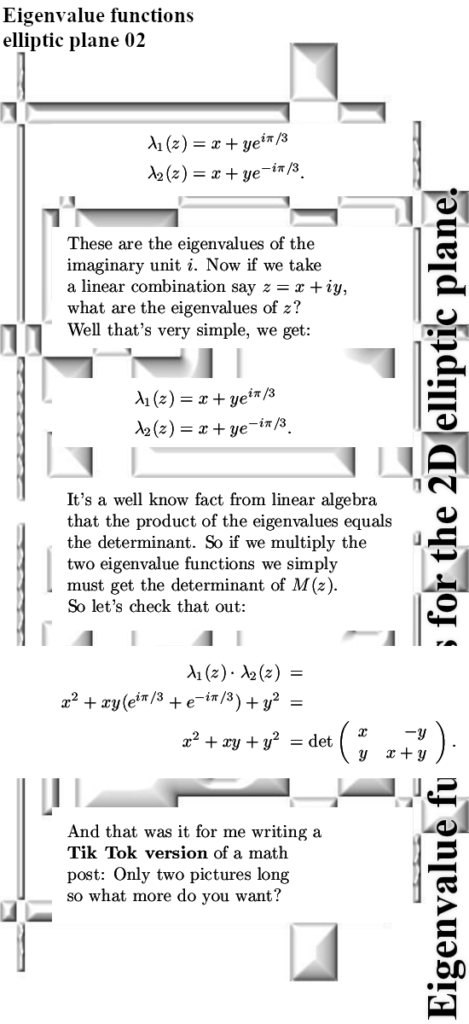
Now I left a lot of stuff out otherwise it would not be a Tik Tok short kind of math post. But you can also use the elliptic complex plane as your primary source of eigenvalues. For example at the other side of our galaxy there lives an alien race known as the Orcs. And for some kind of religious reason these Orcs just don’t want to use circles because as they all know circles are evil. But they found the elliptic complex plane and they use that for solving eigenvalue problems like eigenvalues from square matrices or even stuff that we humans know as the Hamiltonian energy operator. That should work just as good as we humans do in using the complex plane we have over here where the complex exponential is a circle.
So let us now look at the third picture that has both factorizations in it:
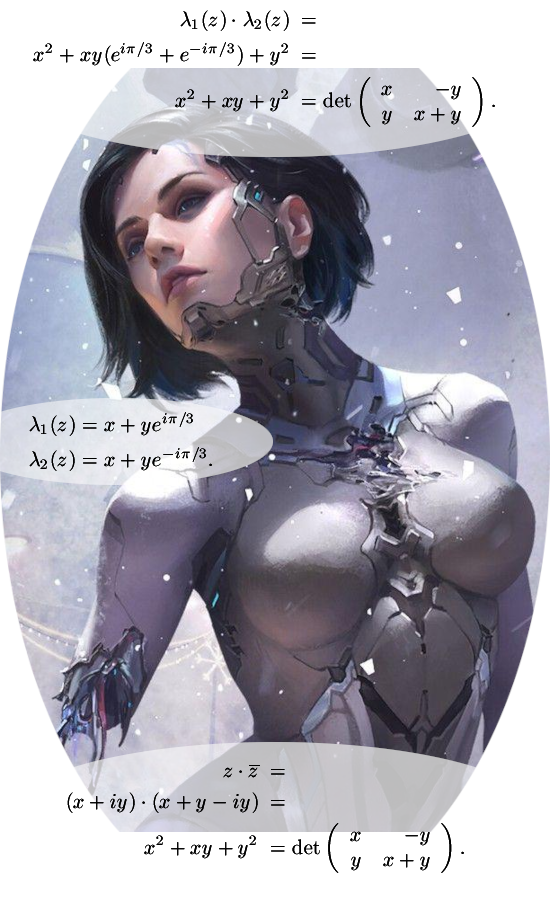
Let me leave it with that and as always thanks for your attention.